Essential minerals fuel battery innovation
Vital minerals in battery cells fuel mobile electrification, requiring manufacturers to maintain sustainable supply chains

Röviden
- Modern batteries are powered by critical minerals. Although lithium is most prominent, others like cobalt, nickel, manganese and graphite are also crucial for battery cathodes, anodes and electrolytes.
- The raw materials used in cells determine batteries’ unique characteristics. However, sourcing minerals for manufacturing often includes economic and environmental challenges and trade-offs.
- Ethical battery production requires responsible sourcing and environmental impact mitigation.
- Battery recycling enables the recovery of valuable minerals from end-of-life batteries, reducing reliance on new mining and mitigating supply chain vulnerabilities.
Mineral-powered mobility
Global energy transition and electrification trends are empowering mobility and electrical adaptability, including electric vehicle (EV) adoption and renewable energy storage solutions. These trends have centered industrial innovators’ focus on battery technology. Driven by steadily increasing demand throughout industry, battery production has surged in recent decades, with new sources of battery minerals and improved efficiencies in manufacturing production processes and technology.
In battery chemistry, the combination of minerals contributes to overall performance. Various elements and compounds are combined to form the electrodes and electrolyte in each cell, with their interactions defining the battery’s characteristics. This page traces the journey of essential minerals from the Earth to batteries that power the mobile and electrical systems the modern world increasingly relies on. It also examines challenges and strategies to maintain supply chain stability.
Insights
Chemistry, electrolyte efficiency, thermal management and charge/discharge mechanisms are the underlying key contributors to battery energy density, power output, lifespan and overall performance.
Raw materials
Lithium-ion (Li-ion) batteries are by far the most prominent type among today’s battery landscape. These assemblies rely on a complex combination of minerals and materials, each contributing to the battery’s unique properties. While namesake lithium is the most prominent elemental component, other minerals are also required to manufacture Li-ion cells.
Cathode materials
A battery’s cathode influences several key performance characteristics, including energy density, power output and cell lifespan.
Valued for its high energy density and stability, cobalt is commonly used in Li-ion battery cell cathodes, especially for EVs. However, cobalt mining faces more ethical concerns than most other battery minerals, requiring responsible battery manufacturers to trace their supply chain origins, while holding upstream stakeholders accountable for sound practices. The European Union, for example, has implemented regulations on what are referred to as conflict minerals. They aim to curb the use of minerals that finance armed conflicts or are mined under conditions that violate human rights.
Nickel is also frequently used in Li-ion cathodes, providing even higher energy capacity by both weight and volume. However, mining nickel raises environmental concerns due to its potential impact on sensitive ecosystems. These effects can include deforestation, habitat loss and water pollution in oceanic regions where the mineral predominantly originates, such as Indonesia and the Philippines. For these reasons, prominent US EV manufacturer Tesla declared its departure from nickel-based Li-ion batteries in the future.
Manganese is more abundant and less expensive than nickel and cobalt, but not as energy dense by weight or volume. However, its lower energy density, makes it less reactive or fire-prone and therefore safer for use in particular Li-ion battery types, such as lithium-manganese phosphate. As a result, power tool producers and other cost sensitive manufacturers often favor this mineral for use in their batteries.
Anode materials
Anodes form the negative electrode of a battery, which are constructed primarily from graphite, a readily available and inexpensive allotrope of carbon. However, graphite mining also raises environmental considerations, primarily potential dust pollution, water contamination and land degradation. Addressing these issues is therefore critical for sustainable operations.
In some newer high-density batteries, the anode is made from silicon instead of graphite due to its ability to store more lithium ions. For the EV industry, this increases range and charging speed. However, silicon's tendency to expand and contract during charging and discharging cycles presents a safety risk that must be mitigated in cell construction.
Electrolyte materials
The electrolyte between a battery’s cathode and anode facilitates ion flow. It is typically comprised of lithium salts dissolved in organic solvents. Lithium hexafluorophosphate, created by reacting lithium fluoride with other solvents, currently dominates the Li-ion electrolyte market, but researchers are also investigating alternative lithium salts and solid-state electrolytes.
Producing lithium
Lithium is most frequently found in South American brine deposits and Australian hard rock formations. It is usually extracted through large-scale evaporation ponds or conventional mining methods. Both extraction approaches require responsible care to minimize harm to local water resources and ecosystems.
Unlike many metals, lithium is not refined into a metallic state, but rather into high-purity soluble compounds, such as lithium carbonate or lithium hydroxide.
Brine extraction
Brine extraction requires concentrating lithium salts from underground brines at concentrations of 200 to 1400 mg/L, typically using large evaporation ponds. Employed at large scale, the process is time-consuming and water intensive.
Once concentrated, the brine undergoes a series of chemical reactions to precipitate out unwanted compounds. This causes eventual crystallization so lithium carbonate can be extracted. Careful monitoring of these reactions and efficient filtration processes are critical to maximize lithium recovery and minimize waste.
Alternatively, direct lithium extraction can be used, providing a more sustainable means of obtaining lithium from brine without the need for evaporation ponds. This process leverages adsorbent materials with an affinity for lithium. It includes clay minerals and ion exchange resins to collect lithium-rich solvents from the brine. Once the adsorbents are saturated with lithium ions, they are subjected to desorption and the lithium solution is collected. Unfortunately, this process is not yet viable at the scale required to meet commercial lithium demand.
Hard rock spodumene mining
Hard rock mining entails quarrying spodumene ore, crushing it and converting it to beta-spodumene through high-temperature heating in rotary kilns. The whole process is quite energy intensive.
Once the ore is converted, it undergoes chemical reactions, such as the brine extraction process, which gradually removes impurities. This continues step by step until only lithium carbonate remains, with minor byproducts at low concentrations. The compound must be further purified by adding a lithium bicarbonate solution, then filtering and reheating it until battery grade lithium carbonate is achieved. This grade is known as “five nines”, or 99.999%, purity.
Insights
Li-ion batteries require 99.999% ultra-pure lithium carbonate, also known as five nines purity.
Refining, manufacturing and assembly
Like lithium, other battery minerals must be refined for purity prior to use in battery cell manufacturing. This typically requires a series of chemical and physical transformations that vary depending on the specific mineral and its intended application. While lithium refining requires multiple stages of purification and filtration, cobalt and nickel are separated through intricate pyrometallurgical or hydrometallurgical processes.
Following refinement, high-purity materials are used to manufacture battery components. Cathode and anode materials are synthesized through precise mixing, heating and coating processes, each requiring reliable measurement and quality control to ensure optimal battery performance.
Electrolytes are formulated by carefully dissolving lithium salts in pure solvents, while restricting moisture intrusion to prevent battery degradation and safety hazards. These components then converge, where they are intricately layered, wrapped and hermetically sealed to prevent leakage and ensure longevity.

During assembly, individual cells are combined into battery packs and modules, tailored for specific applications including smartphones, EVs and sophisticated battery-backed power management systems.
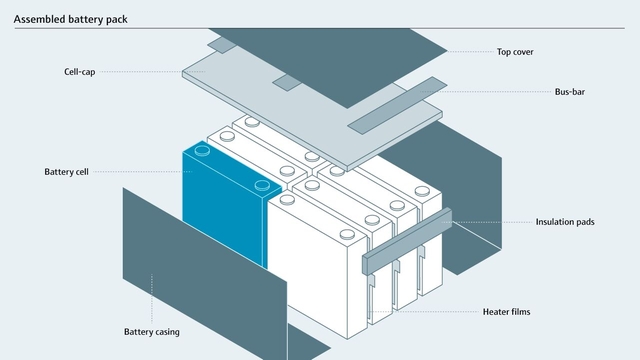
Recycling and circular economy
The journey of battery minerals doesn’t end with their first use. As industry and the public recognize the finite nature of these resources and the resulting environmental impact from their extraction and processing, there is a push to embrace the principles of circular economy. Achieving this requires efforts to enhance battery recycling viability, enabling the recovery of valuable minerals from end-of-life batteries. This reduces reliance on new mining and mitigates supply chain vulnerabilities.
Sustaining the battery supply chain
As demand for lithium-ion batteries surges, optimizing mineral extraction, production and recycling processes is imperative to ensure ongoing sustainable operations and minimize environmental harm. Measures for consideration include implementing robust water management strategies, adhering carefully to regional environmental regulations and investment by mining companies and stakeholders in direct lithium extraction.
Battery mineral production represents the interconnected nature of technology, environment and societal movement. As humanity navigates the great energy transition and collectively progresses toward carbon-neutral goals, industry must integrate ethics, environmental protection and profitability into its long-term sustainability strategies. Ongoing success requires technological advancement, ethical sourcing of raw materials and overall sustainable production.